Phase-transfer catalysis excels in transferring and reacting all kinds of oxidizing agents including permanganate and dichromate. Permanganate has a very high affinity for quaternary ammonium cations that enhances the extractability of this anionic oxidant. The first publication that launched the field of PTC, authored by Charles Starks, explicitly cited the use of Aliquat 336 with potassium permanganate (taught on page 197 of our PTC course manual). Another of the earliest PTC publications in the 1970’s was entitled “Purple Benzene” to describe what happens when you mix a quaternary ammonium salt with potassium permanganate and benzene (yes…we used benzene as a solvent in the 70’s!).
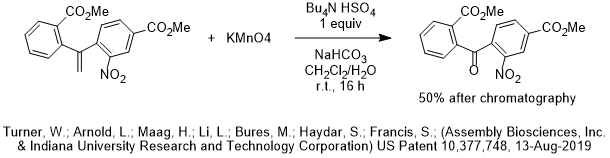
We don’t often see patents recently that cite the use of PTC with permanganate, but this August 2019 patent shows the reaction in the diagram that oxidizes a geminal alkene to a ketone using bicarbonate for pH control. A full equivalent of tetrabutylammonium hydrogen sulfate was used instead of catalytic quantity and it is not clear why so much was chosen to be used by the inventors.
PTC-permanganate oxidation of alkenes can produce a variety of products including diols, aldehydes, ketones, carboxylic acids and hydroxy-ketones depending on [1] the structure of the substrate [2] the pH of the aqueous phase in liquid-liquid PTC-permanganate systems and [3] and whether using aqueous workup or dry workup for solid-liquid PTC-permanganate systems. For more details, contact Marc Halpern of PTC Organics to explore PTC consulting to achieve your company’s process development goals.
One challenge of the use of PTC-permanganate reactions for commercial applications is the formation of manganese dioxide precipitate that is difficult to filter as a finely divided solid.
A patent was issued this month that used tetrabutylammonium bromide as a bromide source that can be used to carefully control at will not only the initiation of the polymerization of propylene oxide or epichlorohydrin, but also control the ratio of repeating units to a cationic end group at only one side of the polymer. This is useful for preparing an effective dispersion of a nanocarbon material in an aqueous phase.
The inventors of the patent Hoang, T.; Ohta, K.; Hayano, S.; Tsunogae, Y.; (Zeon Corporation) US Patent 10,344,124, 09-Jul-2019, found that a nanocarbon material is more favorably and more stably dispersed in a polyether-based polymer that contains a cation on only one terminal side. Examples of such polyethers that contain a cation on one side are polypropylene oxide or polyepichlorohydrin that has an onium salt on one side that can be produced by reacting an amine or a nitrogen heterocycle with a polypropylene oxide or polyepichlorohydrin that has a bromide at only one end.
For this purpose, in one example the inventors polymerized propylene oxide in the presence of a carefully chosen amount of tetrabutylammonium bromide (TBAB) as an organic soluble bromide source (21.5:1 molar ratio of PO:TBAB), triethylaluminum as polymerization catalyst and toluene as the solvent at 0 C for 2 hours. When the reaction was complete, the inventors added isopropanol to terminate the reaction and obtain polypropylene oxide with an average of 20 PO units, having a bromomethyl group at the polymerization starting terminal and a hydroxyl group at the polymerization terminating terminal.
The polypropylene oxide with bromomethyl at one end and hydroxyl at the other end was quaternized with 1-methyl imidazole or butyl dimethyl amine.
In other examples, the inventors reduced the amount of TBAB which increased the number of PO repeating units to 50, 100 and 200 at will and by design, in order to test for the optimal chain length for the stable dispersion of the nanocarbon material.
While this application is not strictly phase-transfer catalysis, it does leverage the concept of controlling a reaction by using a phase-transfer agent to introduce an anion into a reaction phase in a specific well-controlled quantity to achieve desired performance targets.
In our 2-day PTC course, we show other examples of using TBAB to initiate nucleophilic reactions induced by bromide that star with ring opening. Now register for the public PTC course in Prague in October 2019 or bring the PTC course in-house to your company to improve your personal performance, your department’s performance and your company’s performance. The increased profit your company will achieve from low-cost high-performance green chemistry using phase-transfer catalysis, may save jobs, perhaps your job.
This PTC Tip of the Month has helped many of our customers save large amounts of money, mostly by reducing excess expensive and/or hazardous raw materials while maintaining high yield and high throughput. The diagram is taken from the group exercise on page 108 of the course manual of “Industrial Phase-Transfer Catalysis.”
This graph shows the disappearance of benzoyl chloride as a function of agitation speed (in a small lab reactor) for the reaction of aqueous sodium phenoxide with benzoyl chloride in the presence of tetrabutylammonium hydrogen sulfate.
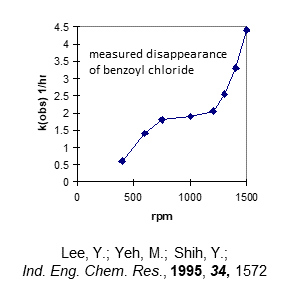
At low rpm, this PTC reaction is transfer rate limited (“T-Reaction”). In the T-Reaction regime, more agitation increases mass transfer of the phenoxide from the aqueous phase into the organic phase and reactivity increases. At some point when increasing agitation efficiency, the rate of consumption of phenoxide in the organic phase by the intrinsic reaction is slower than the rate of phenoxide transfer. In other words, the rate determining step shifts from mass transfer (“T-Reaction”) to the intrinsic nucleophilic substitution in the organic phase (“I-Reaction”).
In the I-Reaction regime, further increase in agitation efficiency does NOT increase throughput since the rate determining step is not no longer limited by transfer.
The sharp increase in the disappearance of the benzoyl chloride at the right side of the graph represents non-catalyzed interfacial hydrolysis of the benzoyl chloride.
In other words, if you think that you must agitate 2 phases as much as possible and you have water-sensitive reactant, all you are doing is causing you purchasing manager to waste money on more benzoyl chloride (in this case).
This exercise is taught in the 2-day PTC course to give you the ammunition to convince your engineers to reduce the agitation efficiency of your 2- or 3-phase PTC systems when you have water-sensitive reactants or products.
Now register for the 2-day course “Industrial Phase-Transfer Catalysis” in order to learn dozens of specialized PTC techniques, like this one, so you can improve your personal performance in process development and plant support. This rare public PTC course (most PTC courses are conducted in-house due to the obvious high value) will be conducted in Prague on October 15-16, 2019 and you can enjoy a 15% discount by registering and paying before June 30, 2019.
Still not sure if you want to attend? Examine the PTC course agenda and you will understand why this is such a powerful course that helps you improve profit performance and R&D efficiency!
This patent describes a high yield PTC C-alkylation performed under dilute liquid-liquid PTC conditions in a microreactor. A forced thin film microreactor (shown in the diagram at https://www.m-technique.co.jp/e/members/ulrea/kouzou.html) is different than many other microreactors. In most microreactors used with phase-transfer catalysis, the two liquids (aqueous phase and organic phase) are fed into in a static channel. In contrast, in a forced thin film reactor, the two liquids (aqueous phase and organic phase) are fed into a narrowing channel in which part of the channel surface is static and the other part of the channel surface is rotating.
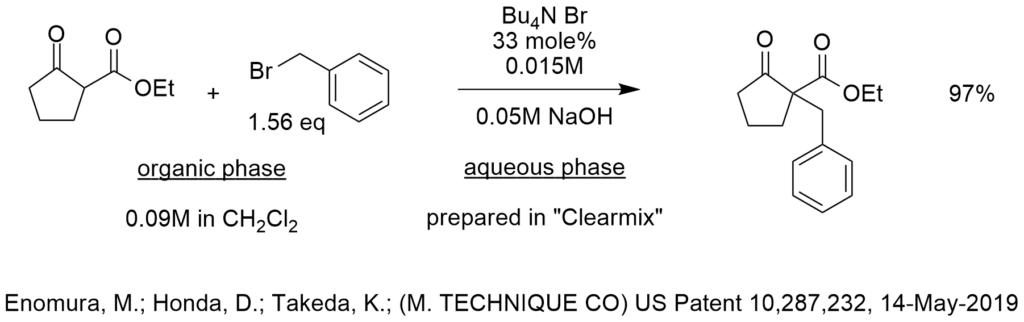
The advantage of the forced thin film microreactor is claimed to be scalability without having to add too many microreactors in series as is commonly done with static microreactors.
For reasons not explained, performance is better when the aqueous phase containing the phase-transfer catalyst and NaOH is agitated for 15 minutes in a “Clearmix preparation apparatus” before being contacted with the organic phase. This seems surprising since the aqueous phase is quite dilute and both the NaOH and TBAB should be dissolved homogeneously without much effort or time. However, the results speak for themselves.
When the aqueous phase is prepared with the Clearmix apparatus (Table 1: Examples 1, 6, 7), the yields are 88% to 97% whereas when the “aqueous solution, which was manually agitated, was used after it was visually confirmed that the reacting agent was dissolved” (Comparative Example 1), the yield was only 51%. In all of these cases, the microreactor in which the PTC reaction was performed was a forced thin film type operating at the same flow rates and same rotation speeds of the rotating surface.
This patent should be studied by those of you who are combining the powerful advantages of phase-transfer catalysis with the powerful advantages of microreactors.
As we teach in our 2-day course “Industrial Phase-Transfer Catalysis” hydration is often the #1 factor that determines the amount of profit generated by a commercial PTC processes. This Dow Corning patent by DePierro and Reisch that issued this month illustrates the high sensitivity of solid-liquid PTC processes to hydration in a narrow range of added water. It is very important to be aware of the sensitivity because too many development teams overlook this important impact on profit when they are not aware of this sensitivity and they either unnecessarily lose yield or experience high variability from batch to batch due to minor changes in moisture level of the solid reactant.
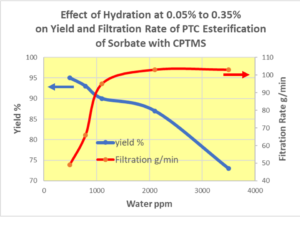
This sensitivity to hydration is sometimes so significant that we have even seen differences in yield, reaction rate, etc. of large scale commercial solid-liquid PTC reactions, between batches when the solid reactant was taken from the middle of a container versus the top of the container that was exposed more to atmospheric moisture.
You really need to be aware of the levels of all hydrogen-bonding species in solid-liquid PTC reactions.
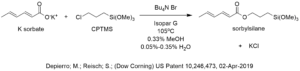
In this case, solid potassium sorbate was reacted with chloropropyl trimethoxy silane (CPTMS) in the presence of 1.7 mole% tetrabutylammonium bromide phase-transfer catalyst, Isopar G as solvent and stabiliziers. The graph shows the yield of the esterified product and the filtration rate after the reaction (to separate KCl byproduct) at a constant level of 3300 ppm methanol. Again, we must be aware of the level of all hydrogen-bonding species.
The inventors were very smart to test the effect of water at various levels up to 0.35% to understand the dramatic impact on yield. The inventors did not report the effect of water on reaction rate though they did mention that the reaction rate varied between 5 hours and 10 hours depending on reaction conditions. “The time required to complete the reaction varied depending on methanol and water concentration as well as temperature.”
Again, we teach process chemists and plant support chemists how to squeeze more profit from commercial solid-liquid PTC processes in our 2-day PTC course or by conducting PTC Process Consulting and PTC Contract Research. Don’t let your company make less profit by hesitating to take our PTC training, PTC Process Consulting or PTC Contract Research. The return on investment when contracting for PTC Organics’ services is often thousands of percent.
Now contact Marc Halpern of PTC Organics to improve process performance and R&D efficiency. The job you save may be your own!
We need your help.
In the late 1970’s, I saw an abstract of a talk at a conference that claimed to use TDA-1 [Tris(3,6-dioxaheptyl)amine], a polyether, to complex with magnesium and improve a Grignard reaction. I never heard the presentation or saw any other reference to it. I let that abstract slip into the depths of my memory.
This month, a procedure was reported in the patent shown in the diagram that uses catalytic TBAB in this Grignard reaction. I am having a hard time speculating the role of the TBAB.
Our subscribers are more knowledgeable and more intelligent than I. I am asking your help to suggest explanations of why the inventors added TBAB to this reaction. I am assuming that is not something they would do if it worked without TBAB.
Please indicate whether we can or should publish your name and company when you submit your suggested explanations.
Now contact Marc Halpern of PTC Organics to help the PTC community understand this reaction.
A patent was just issued to Ultraclean Fuel with Gordon Gargano and Marc Halpern as the inventors that describes the large scale desulfurization of fossil fuel hydrocarbons to produce “ultralow sulfur diesel” (ULSD) and other ultralow sulfur hydrocarbon products that contain less than 10 ppm sulfur. These outstanding results require the use of a carefully selected phase-transfer catalyst, a phosphotungstate co-catalyst and hydrogen peroxide to oxidize covalently bound sulfur to sulfones. The sulfones are removed from the hydrocarbon stream to produce high quality desulfurized product. The patent reference is Gargano, G.; Halpern, M.; (Ultraclean Fuel Pty Ltd) US Patent 10,214,697, 26-Feb-2019.
Process equipment is described (Figures 4-6) that can process 1,500 barrels per day of high sulphur hydrocarbon feed (63,000 gallons) with the potential for 15,000 barrels per day (630,000 gallons).
This patent is a follow up to US Patent 9,441,169 by Gargano and Halpern.
The impressive breakthrough results are as follows:
“Transmix/Diesel feed containing a total sulphur level of 271 ppm was successfully desulphurised to a total sulphur level of 0 ppm according to ASTM D5623 standards and from 334 ppm to 2 ppm according to ASTM D5453 standards.”
“Transmix Diesel Hydrocarbon containing a total sulphur level of 407 ppm was successfully desulphurized to a total sulphur level of 9.2 ppm.”
“Refinery Diesel Hydrocarbon containing a total sulphur level of 3996 ppm was successfully desulphurized to a total sulphur level of 10 ppm.”
“Natural Gas Condensate containing a total sulphur level of 432 ppm was successfully desulphurized to a total sulphur level of 4.8 ppm.”
“Jet Fuel containing a total sulphur level of 1518 ppm was successfully desulphurized to a total sulphur level of 9 ppm.”
This patent is a good example of achieving a major process breakthrough by integrating the highly specialized expertise of Marc Halpern of PTC Organics with the highly specialized expertise of a company in their primary field of focus. Your company should similarly consider achieving major breakthrough process technology to achieve best in class competitive advantage.
Now contact Marc Halpern of PTC Organics to inquire about collaboration to improve your company’s profit and achieve low-cost high-performance green chemistry.
Before reading this patent, please be aware that PTC Organics developed PTC etherification technology using epichlorohydrin that is more selective than that reported here and in other PTC glycidyl etherification literature. PTC Organics maintains this technology under trade secret status. Contact Marc Halpern of PTC Organics to inquire about licensing PTC etherification technology using epichlorohydrin.
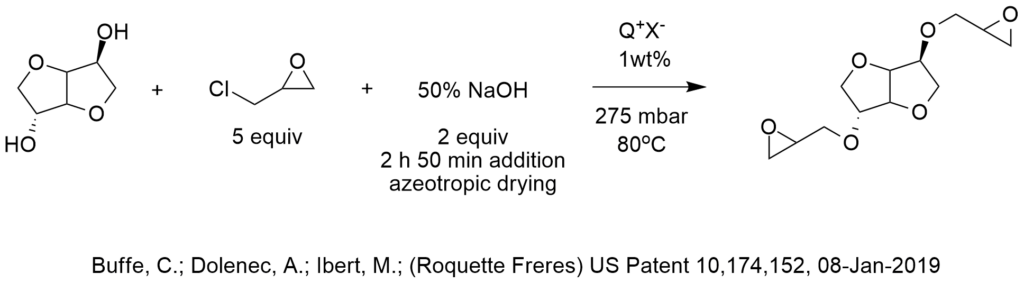
One of the largest scale early commercial applications of phase-transfer catalysis was the reaction of bisphenol A with epichlorohydrin to for the bisphenol A-diglycidyl ether (DGEBA), a monomer used in epoxy resins. The inventors note that isosorbide diglycidyl ether may be a suitable substitute for DGEBA which has raised concerns about carcinogenicity even though there are no data that support human carcinogenicity. This patent describes the use of PTC for the etherification of isosorbide (derived from a natural product) with epichlorohydrin.
The challenge is to selectively produce the diglycidyl ether while minimizing both residual monoglycidyl ether and higher oligomers that result from hydrolysis of the epoxide and subsequent oligomerization. The inventors found that using 1 wt% tetraethylammonium bromide or tetrabutylammonium iodide maximizes the desired digylcidyl ether.
The inventors used 5 equiv epichlorohydrin. This level of excess is common in reports of other glycidyl etherifications. The excess epichlorohydrin also provides extra fluidity since no additional solvent is used.
50% NaOH (2.0 equiv) was added over time with continuous azeotropic drying to minimize hydrolysis, to neutralize the 2 hydroxyls without excess hydroxide.
The reaction was performed at 80 C. This is also a common temperature used in the literature foe etherifications using epichlorohydrin.
The attention to detail in reporting by the writers and examiners of the patent is poor. In all the examples, the identity of the phase-transfer catalyst quat is referred to as triethylammonium instead of tetraethylammonium and the abbreviations for the quats in the table are amazingly: TBAI = triethylammonium iodide, TEAC = triethylammonium chloride and TEAB = triethylammonium bromide. Look it up if you have a hard time believing that such errors would make it through all the reviews. We know that the real catalysts are tetraalkyl quats based on the claims, which apparently were actually read before issuing the patent.
If your company has a commercial application that can greatly benefit from selective PTC etherification technology, especially using epichlorohydrin, now contact Marc Halpern of PTC Organics to explore business opportunity under secrecy agreement.
One of the major advantages of phase-transfer catalysis is the ability to replace expensive anhydrous bases with PTC and common inexpensive bases. There are countless examples of reports in which chemists use t-butoxide when they could and should use PTC-NaOH or PTC-potassium carbonate.
However, there are few examples when chemists are clever enough to think about using PTC but continue to use t-butoxide in cases that don’t require deprotonation of high pKa substrates. This patent is such an example.
In fact, the reactant being deprotonated is a phenol which is so acidic that it doesn’t even need NaOH, let alone t-butoxide. Potassium carbonate by itself is basic enough to deprotonate the phenol. Potassium carbonate can also serve as a desiccant to “hide” any water in the system. This is important because nucleophilic aromatic substitutions usually need significant energy of activation and we don’t want to add energy of activation due to hydration of the attacking phenoxide anion.
Moreover, I would be concerned using excess t-butoxide that can cause Hofmann Elimination of the TBAB that decomposes the phase-transfer catalyst, especially at 80-85 C. Who knows…maybe that is why the inventors used such a high catalyst loading of 20 mole%, to overcome catalyst decomposition.
It is always possible that the inventors tried PTC with just carbonate (no t-butoxide) and it didn’t work. However, I would be surprised if that was the case.
If your company is using t-butoxide or any other expensive, anhydrous and/or hazardous base, now contact Marc Halpern of PTC Organics to reduce your cost of manufacture, reduce your development time and improve process performance by integrating PTC Organics’ highly specialized expertise in industrial phase-transfer catalysis with your process development and commercial goals.
In the October 2018 PTC Reaction of the Month, we asked for suggestions to explain the mechanism for the tetramethylammonium chloride catalyzed esterification of methacrylic acid with epichlorohydrin. Peter Kapferer, a process chemist at a well-known manufacturer in Switzerland, proposed the following mechanism that is quite plausible.
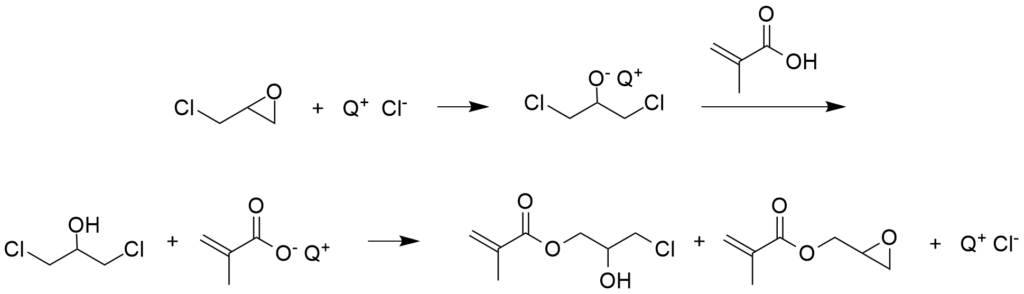
Peter wrote: Opening of the epoxide by the Quat-chloride would, in the absence of any inorganic cations, lead to Quat-1,3-dichloro-2-propanolate, a strong base. This could take the proton from methacrylic acid, and then Quat-methacrylate reacts with Quat-1,3-dichloro-2-propanol to form the ester and Quat-Cl, closing the catalytic cycle. There are a few examples in the literature where tertiary amines and an epoxide form strong alkoxide bases. The advantage of the two-stage process could thus be to carry out the esterification under more or less neutral conditions (with only traces of a base formed in-situ), avoiding the formation of oligomers by repeated addition of epichlorohydrin. As the ester forms, the base “disappears”.
We thank Dr. Kapferer for this contribution and continued interest in the PTC Tip of the Month for more than a decade.
We also corrected the name of the inhibitor shown in the diagram in the October 2018 PTC Reaction of the Month,