I invented the empirical “q-value” parameter when I was too lazy to work in the lab one day in 1988. At that time, we just received a new MacIntosh computer that had CricketGraph installed and it had the amazing capability to draw a graph from data, something that my Lotus 1-2-3 software on my Leading Edge Model D at home could not yet do, even though it had dual 5.25” floppy drives and upgraded whopping 256 KB RAM.
Now might be a good time to get a cup of coffee to read the story of the “Halpern q-value” which I hope you will find to be at least mildly interesting, if not entertaining and of course valuable from a scientific standpoint. Spoiler alert: this story will end with an article that I just learned has an alternative explanation of the q-value. That article by Denmark and Henle from 2015 has excellent correlations and excellent suggestion for underlying fundamentals…something that has been missing from my empirical q-value parameter for decades.
For several years in the 1980’s, I was looking for a way to EMPIRICALLY represent an asymptotic diminishing effect of the number of carbons of an alkyl group as a predictor for reactivity of interfacial PTC reactions (later refined to be transfer-rate limited PTC reactions – “T-Reactions” – in discussions with the superstars Charles Starks and Charles Liotta in Starks’ penthouse in Tulsa, Oklahoma in 1991). In the early 1980’s, I found that many PTC-NaOH reactions, that behaved very differently than almost all other non-NaOH PTC reactions, gave unusually high reactivity as the number of carbon atoms DECREASED for at least one of the four alkyl chains of a quaternary ammonium phase-transfer catalyst, with extra special dominant effect of the length of the shortest alkyl chain on the quat cation as long as there were at least 8-10 carbon atoms total on the quat. Quats such as tetraethyl ammonium (8 carbon atoms total) were usually totally useless for almost all non-NaOH PTC reactions but worked great for PTC-NaOH reactions. This was the basis for my Ph.D. thesis in 1983 (“Phase-Transfer Catalysis of Hydroxide Ion Initiated Reactions: Mode of Action and Applications”) and my first 15 publications before leaving graduate school. In particular, I found that methyl tributyl ammonium was an outstanding phase-transfer catalyst for PTC-NaOH reactions, though the reviewers of Tetrahedron Letters didn’t think this merited publication (a psychological blow in my early years since they published almost anything!). Years later, I had no trouble convincing chemical companies to use hundreds of tons per year of this phase-transfer catalyst in single processes due to multiple factors of excellent performance, but I am getting ahead of my story.
By 1979 (1st year graduate student), I speculated that the accessibility of the positive charge on the nitrogen of the quat might be a plausible factor for affecting reactivity, especially due to the very pronounced high reactivity of nonsymmetrical quats with one methyl group or three ethyl groups. For example, in the alkylation of deoxybenzoin (pKa 16), the order of quat reactivity was methyl tributyl ammonium > ethyl tributyl ammonium > tetrabutyl ammonium > octyl tributyl ammonium. The alkyl trioctyl ammonium series behaved in the same exact way, except that the alkyl tributyl ammonium curve was higher in reactivity than the alkyl trioctyl ammonium curve. That is how I came up with idea of “accessibility” of the positive charge on the nitrogen atom as a likely factor that affects reactivity in many, though not all PTC-NaOH reactions, that happened to make up about 60% of commercial PTC applications at that time.
The raw data are shown in Figure 1 in the bottom 2 curves “c” and “d” (scanned from my Ph.D. thesis…hence the Hebrew figure title).
Figure 1: Quat Structure Effect on Reactivity (bottom graphs “c” and “d”) and O-/C-Selectivity (upper graphs “a” and “b”) in the Alkylation of Deoxybenzoin
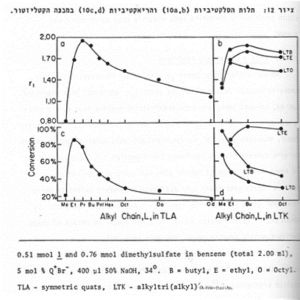
There was something special about a quat with one methyl group (together with 3 alkyl groups with C4 or higher) or three ethyl groups, that induced extra high reactivity in PTC-NaOH reactions.
I looked at a variety of potential metrics and correlations without success from 1979 to 1983 (at which time I left graduate school to start my career). These attempts included looking at bathochromic shifts in the UV of quat picrates and C-13 NMR behavior of the carbon in quat thiocyanates. Eventually, I gave up…
…until that day of lab laziness in 1988 with a brand new Mac on my desk that had the first hard drive I ever saw (a massive tower with an amazing 20 MB of storage capacity!).
In that moment, it all of a sudden hit me that the ridiculously simple arithmetic function of the reciprocal might do the trick since the difference between 1/1 and 1/2 was big, between 1/2 and 1/3 smaller, between 1/3 and 1/4 even smaller and between 1/10 and 1/11 made almost no difference. After all, Coulombs Law tells us that the attraction force between two charges drops off rapidly with the square of the distance between them, so it was not unreasonable to think that accessibility of the positive charge on the nitrogen made a difference on ion pairing. So, I simply added the four reciprocals of each of the four alkyl chains of each of 16 quats, both symmetrical and nonsymmetrical, and I plotted them against the reactivities I found for the alkylation of deoxybenzoin that I measured 9 years earlier (just before I met my wife with whom I am soon to celebrate our 40th wedding anniversary). Lo and behold, 15 of the 16 points lined up in almost a straight line.
Just as I hit “Enter” when creating the Cricket Graph plot of the reciprocal sums versus the reactivities of these 16 reactions, the cleaning lady walked in when I screamed out loud something along the lines of “holy excrement!” The cleaning lady asked if I was OK and I showed her the approximate straight line (except for tetramethylammonium) while excitedly communicating that I have been looking for such a correlation for the better part of a decade. She did not share my enthusiasm and left shaking her head about these crazy scientists.
I was excited because this was the first time anyone ever came close to making sense for correlating or even predicting reactivity simultaneously for both symmetrical and nonsymmetrical quat cations. To this day, I have not seen other attempts to quantifiably correlate quat structure with reactivity for both symmetrical and nonsymmetrical quats. In fact, whenever I teach this industrial PTC course, the #1 comment I get in the evaluation forms is that the participants were not aware that there is a systematic method to evaluate and optimize PTC applications nor did they know that the Halpern q-value was a part of that valuable thought process that minimizes the number of experiments required to develop and optimize PTC applications under industrial process development project pressures and deadlines.
This is why I recently presented the new lecture “How to Improve Process R&D Efficiency Using Phase-Transfer Catalysis” that is ow available for free viewing for a limited time at https://vimeo.com/421146888.
In any case, the original graph that correlated q-value with the reactivity of the alkylation of deoxybenzoin is shown in Figure 2, which is a page from my course “Industrial Phase-Transfer Catalysis” exactly as it has appeared in 56 sessions of this course since 1996. Figure 2 also shows how the Halpern q-value is first introduced during the course as an “empirical parameter.”
Figure 2: The Halpern q-value correlation for Reactivity of the PTC Alkylation of Deoxybenzoin from the Industrial PTC Course Manual
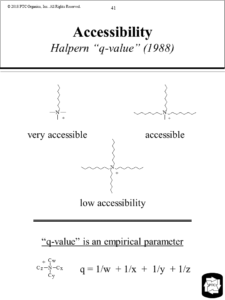
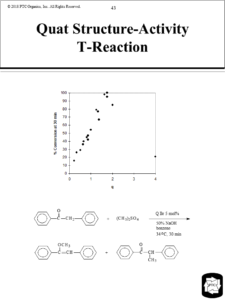
Apparently, I exhausted all my creative ability that day in coming up with the notion of adding four reciprocals, so I non-creatively named this empirical parameter “q-value” wherein “q” referred to some property of a “quat cation.”
I immediately knew that the q-value was nothing more than an empirical parameter. I presented the q-value to Starks and Liotta who I first met a couple of years earlier in December 1986 at a PTC summit at Catalytica in Silicon Valley, due to the efforts of Howard Alper and David Hamm.
We could not figure out a rational explanation for why the q-value worked and I was encouraged when Charles Liotta (absolutely brilliant scientist with an amazing sense of humor, outstanding interpersonal skills and a master at ballroom dancing!) said that the q-value was probably a valid parameter to consider. Starks and Liotta were my greatest mentors.
Starks, Liotta and I conducted a few sessions of a 3-day PTC course for process chemists in the US and the UK between 1990 and 1995. Whenever I presented the q-value, I always stated that it was nothing more than an empirical parameter and any of you who have my PTC course manual (I conducted the 2-day version of the industrial PTC course 56 times from 1996 to 2019) can look up in the manual and see that I refer to the q-value as a strictly empirical parameter.
At the same time, since the dominance of one methyl group or three ethyl groups on the quat, affects reactivity so much, I have always stated that until and unless a better explanation is offered, I will refer to the q-value as a metric of accessibility of the positive charge on the nitrogen of a quat.
In fact, Figure 3 shows that methyl trioctylammonium gives 70% less reactivity than tetrahexylammonium in the PTC-NaOH isomerization of allylbenzene which is an “I-Reaction” (not a T-Reaction). Since these two quats have about the same number of carbons atoms (25 vs 24) and methyl trioctylammonium dissolves fully in the solvent toluene, a plausible explanation in 1983 was that the methyl trioctyl ammonium cation had a more accessible positive charge on the nitrogen atom than tetrahexyl ammonium and the tighter ion pair reduced reactivity. This is just one of several examples shown in my course “Industrial Phase-Transfer Catalysis” in which PTC I-Reactions go much slower with more accessible quats with the same number of atoms, such as decyl triethyl ammonium versus tetrabutylammonium, both with 16 carbon atoms but radically different accessibilities of the positive charge. I still think that ion pairing is very important and that q-value is useful as a predictor for reactivity in PTC I-Reactions, especially when comparing both nonsymmetrical quats and symmetrical quats for a single reaction. Afterv all, in the real world, we do not limit ourselves to just symmetrical quats.
Figure 3: Methyl Trioctyl Ammonium Shows Greatly Decreased Reactivity Relative to Tetrahexylammonium for a PTC I-Reaction Even Though They Have Nearly the Same Number of Carbon Atoms…Likely Accessibility of the Positive Charge Affects Ion Pair Tightness
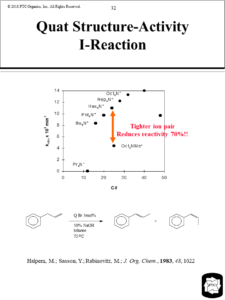
A couple of weeks ago, I happened to stumble across a paper (Denmark, S.; Henle, J.; Chem. Sci., 2015, 6, 2211) that addresses the underlying fundamentals of the q-value instead of just stating the empirical correlation between q-value and reactivity for PTC “T-Reactions.” This article is VERY worthwhile reading.
The authors found excellent correlations between reactivity of one of the classical PTC T-Reactions (an O’Donnell C-alkylation) and the “quaternary ammonium cross sectional area (XSA) as a general descriptor for transport-limiting PTC rate approximations.”
One of the strengths of the empirical q-value that has served me well over the past 3 decades has been the ability to compare the wide array of commercially available quat salts, INCLUDING NONSYMMETRICAL quats, for the optimization of large scale commercial PTC processes that has saved our customers and commercial partners hundreds of millions of dollars. The Chem Sci paper cited above refers only to symmetrical quat cations. So, while the Halpern q-value may not be a philosophically accurate concept for accessibility, it remains a very valuable empirical predictor of real world performance for real world quats, including nonsymmetrical quats such as methyl tributyl ammonium, Aliquat 336 and Adogen 464.
When I entered into the field of PTC in 1976 as a 2nd year undergraduate student, 5 years after the publication of Starks’ classical 1971 paper that first disclosed the term “phase-transfer catalysis,” the undeniable difference in quat structure-activity relationships between almost all PTC-NaOH reactions and almost all non-NaOH PTC reactions was noticed but not discussed in the open literature. When I performed the study in 1980 of the kinetics of the isomerization of allylbenzene using PTC-NaOH conditions (Figure 3 above), I was shocked to obtain perfect pseudo-1st order kinetics and higher reactivity with more organophilic quats, just as observed for almost all non-NaOH PTC systems. That paper was published in 1983 (Halpern, M.; Sasson, Y.; Rabinovitz, M.; J. Org. Chem., 1983, 48, 1022) and is probably the paper with the most consistent kinetic behavior through 6 half-lives for a PTC-NaOH system.
Here we are, 50 years after the coining of the term “phase-transfer catalysis,” and we still don’t have a full understanding of the mechanism of the most widely used commercial PTC applications which are PTC-hydroxide systems. Nevertheless, I am glad that many process chemists have been able to leverage the empirical Halpern q-value over several decades to achieve low-cost high-performance green chemistry to create/save jobs and help the environment, including using nonsymmetrical quats such as methyl tributyl ammonium chloride, that has a q-value of 1.75 (and used to be called Aliquat 175 by Henkel-Cognis-BASF when I helped them market that catalyst).
If your company wants to benefit from the most highly specialized expertise in industrial phase-transfer catalysis to achieve low-cost high-performance green chemistry while saving jobs (maybe yours!) and while helping the environment, now contact Marc Halpern of PTC Organics Inc to explore integrating this highly valuable well-seasoned expertise with your commercial goals in the most practical and operational manner.
Figure 4: Marc Halpern (long hair on the right) and Professor Mordecai Rabinovitz at Marc Halpern’s First Poster Session In Jerusalem circa 1980 (the letters “PTC” are visible…a model of QX transfer using volumetric flask caps on a circular track between a blue aqueous phase and a red organic phase is hanging from the bottom of the poster!) My body mass has increased roughly 50% since that picture was taken and it’s not muscle!
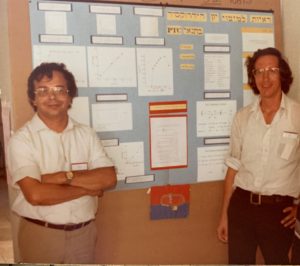